Electromagnetism is one of the four fundamental forces of nature, integral to both theoretical physics and everyday engineering. From the generation of electricity to the transmission of data through fiber optics and wireless networks, electromagnetic principles are at the core of nearly every modern technological system.
This article provides a concise yet comprehensive overview of electromagnetism — its physical foundations, governing equations, and applications — written with the technical depth expected by engineers and advanced learners.
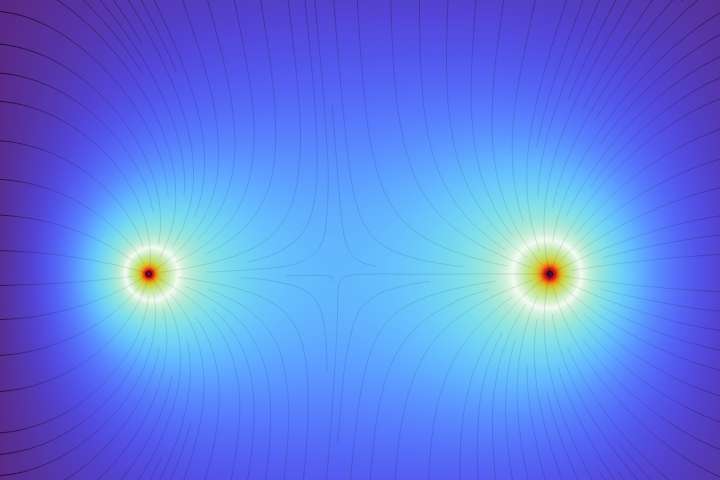
Electromagnetism is a branch of physics that studies the interaction between electric charges and magnetic fields. It is fundamental to our understanding of the physical universe and has led to transformative technologies in communication, medicine, energy, and transportation. The backbone of classical electromagnetism is described by Maxwell’s equations, which unify electricity and magnetism into a single framework. These four partial differential equations describe how electric and magnetic fields originate and evolve due to charge distributions, electric currents, and time-varying fields.
The four Maxwell's equations in differential form are:
$$\begin{aligned}
\nabla \cdot \mathbf{E} &= \frac{\rho}{\varepsilon_0} \\
\nabla \cdot \mathbf{B} &= 0 \\
\nabla \times \mathbf{E} &= -\frac{\partial \mathbf{B}}{\partial t} \\
\nabla \times \mathbf{B} &= \mu_0 \mathbf{J} + \mu_0 \varepsilon_0 \frac{\partial \mathbf{E}}{\partial t}
\end{aligned}$$
These laws collectively explain the behavior of electric fields ($\mathbf{E}$), magnetic fields ($\mathbf{B}$), electric charge density ($\rho$), and current density ($\mathbf{J}$). Gauss’s Law describes how electric charges produce electric fields. Gauss’s Law for Magnetism asserts that magnetic monopoles do not exist—magnetic field lines always form closed loops. Faraday’s Law shows that a time-varying magnetic field induces an electric field, which is the fundamental principle behind electric generators and transformers. Finally, Ampère’s Law (augmented by Maxwell’s displacement current term) reveals how magnetic fields are generated by both electric currents and time-varying electric fields.
A remarkable result of Maxwell’s equations is the prediction of electromagnetic wave propagation. By manipulating these equations in the absence of sources ($\rho = 0$, $\mathbf{J} = 0$), one obtains wave equations for both $\mathbf{E}$ and $\mathbf{B}$ fields:
$$
\nabla^2 \mathbf{E} - \mu_0 \varepsilon_0 \frac{\partial^2 \mathbf{E}}{\partial t^2} = 0, \quad \nabla^2 \mathbf{B} - \mu_0 \varepsilon_0 \frac{\partial^2 \mathbf{B}}{\partial t^2} = 0
$$
These equations describe electromagnetic waves traveling at the speed of light $c$, where $c = \frac{1}{\sqrt{\mu_0 \varepsilon_0}}$. Thus, light itself is an electromagnetic phenomenon, a discovery that unified optics with electromagnetism in the 19th century.
🎥 Want to See Electromagnetism in Action?
If you’ve made it this far, you’ve got a solid understanding of how electromagnetism works on paper. But if you're curious how this theory plays out in real-world simulations, here’s something you’ll appreciate:
👉 Permanent Magnet Simulation in COMSOL – Complete Tutorial
In this video, we walk through the setup of a magnetic simulation using COMSOL Multiphysics. It’s a hands-on example of how to model permanent magnets, visualize magnetic flux lines, and interpret the results — all using a real-world tool that engineers and researchers actually rely on.
You’ll see:
- How to define magnetic materials
- What magnetic field lines actually look like in 2D and 3D
- And how boundary conditions and meshing affect the results
If you’re an engineer, student, or just someone who wants to see theory come to life, this is worth a watch. It bridges the gap between equations and actual design.
Applications in Engineering
Power Generation and Transmission
Faraday's law is the foundational principle behind generators and transformers. Rotating a coil within a magnetic field induces a current — a principle used extensively in power plants.
Electromagnetic Motors
DC and AC motors convert electrical energy into mechanical torque using Lorentz forces acting on current-carrying conductors within magnetic fields. Luckily, I also have a video showing how to model permanent magnet and related physics in COMSOL .
Telecommunications
All wireless communication (Wi-Fi, 5G, Bluetooth) uses modulated electromagnetic waves. Antenna design and impedance matching rely heavily on Maxwellian theory.
EM Shielding and Compatibility
Engineers must consider electromagnetic interference (EMI) in system design — especially in high-frequency circuits and aerospace applications.
Medical Imaging
Magnetic Resonance Imaging (MRI) uses nuclear magnetic resonance, a quantum mechanical effect, to visualize soft tissues by aligning hydrogen nuclei with strong magnetic fields.
In modern applications, electromagnetic theory is not just theoretical—it is embedded in practical devices such as electric motors, inductors, capacitors, waveguides, transmission lines, transformers, and wireless antennas. The equations are often too complex to solve analytically for real-world geometries, which is where numerical simulations come in. Techniques such as Finite Difference Time Domain (FDTD), Finite Element Method (FEM), and Boundary Element Method (BEM) allow engineers and physicists to model electromagnetic field behavior in intricate structures. For instance, the design of a 5G antenna array or an MRI scanner coil requires precise electromagnetic field control, which is only feasible through simulation software like ANSYS HFSS, CST Studio Suite, or COMSOL Multiphysics.
Consider the case of a high-frequency resonant cavity used in particle accelerators. These cavities are carefully shaped metallic chambers where electromagnetic waves resonate to accelerate charged particles to near-light speeds. The performance of such a cavity depends on its ability to maintain strong, well-confined electric fields without excessive energy loss due to resistive heating or radiation. Researchers simulate the eigenmodes of such cavities using FEM-based tools to determine the optimal shape, material, and mode of operation. Detailed experimental verification is then conducted, matching simulation data to real-world results.
In medical imaging, magnetic resonance imaging (MRI) systems use powerful magnetic fields and pulsed radiofrequency (RF) fields to excite hydrogen nuclei in the human body. The resulting emitted signals are captured and converted into detailed anatomical images.
Recent research in electromagnetism has yielded significant advancements across various domains. Below are some notable developments presented in paragraph format:
Researchers at Rice University have made strides in understanding magnetism’s role in quantum technologies. By analyzing FeSn thin films with techniques like molecular beam epitaxy, they identified how localized electrons drive selective band renormalization, a finding with implications for high-temperature superconductors and topological quantum computing. This work is detailed in their October 2024 study.
A groundbreaking discovery in magnetism—termed altermagnetism—was experimentally confirmed using spin-resolved photoemission spectroscopy. This third branch of magnetism, observed in materials like manganese telluride, exhibits unique spin symmetries that could revolutionize spintronics and superconductivity research. The findings, published in February 2024, highlight its potential for next-gen memory devices.
In Earth sciences, advancements in electromagnetic induction methods are reshaping planetary studies. Modern techniques like airborne and marine controlled-source electromagnetics now enable deeper insights into geomagnetic currents and subsurface structures, as outlined in a 2024 review. These interdisciplinary efforts underscore electromagnetism’s expanding role in both fundamental science and technological innovation.
Electromagnetism is not just a theoretical framework but a living, evolving toolset that powers our modern world. From predicting how fields evolve in time and space to modeling real-world devices and systems, its reach is broad and impactful. The precision and efficiency of these systems—be it in communication, energy harvesting, medical diagnostics, or high-frequency electronics—depend heavily on both a strong theoretical foundation and advanced numerical simulations.
Official Links of some of the tools mentioned:
Discussions? let's talk here
Check out YouTube channel, published research
you can contact us (bkacademy.in@gmail.com)
Interested to Learn Engineering modelling Check our Courses 🙂
All product names, trademarks mentioned in this article are the property of their respective owners. This content is for educational purposes only and is not affiliated with, endorsed by, or sponsored by any software company. For official information, please visit the respective official websites. The views expressed are those of the author ONLY.